Your cart is currently empty!
Are We Living in a Simulated Universe? A Comprehensive Exploration
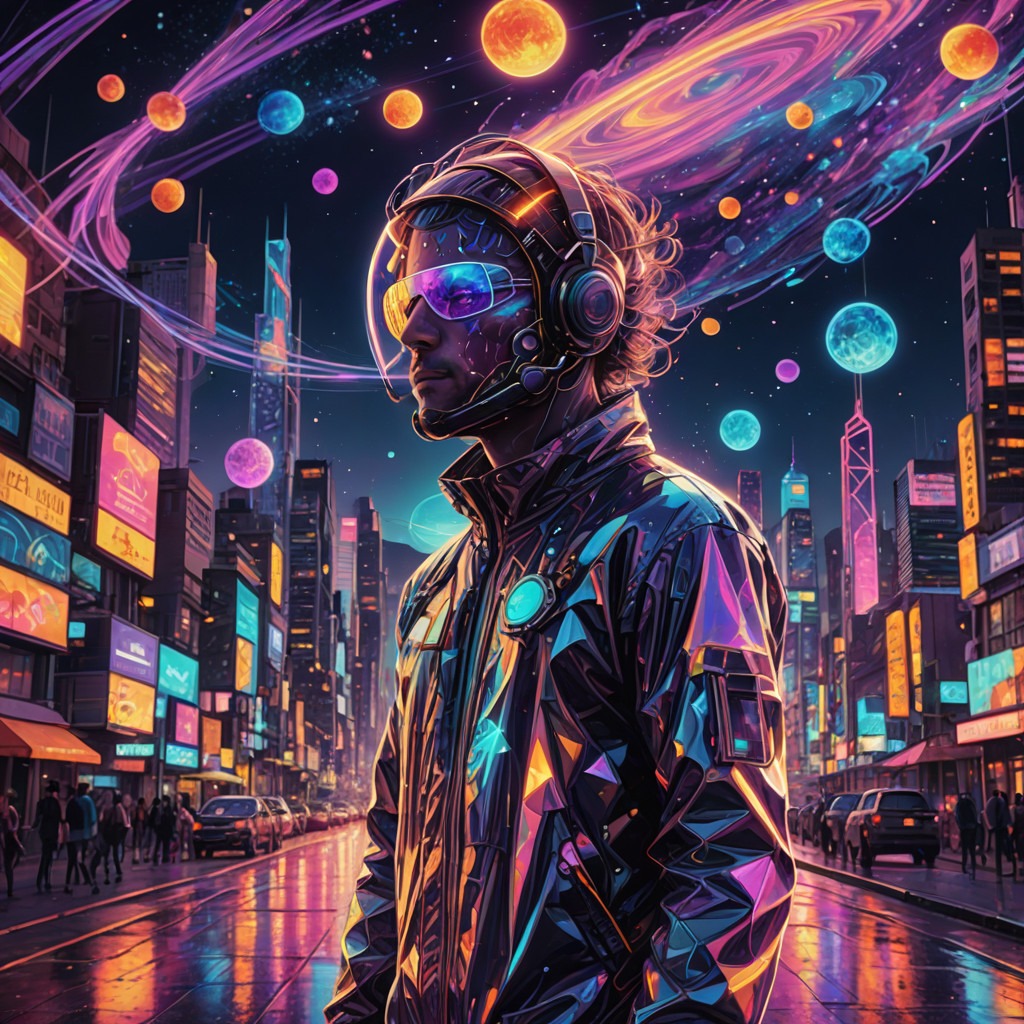
Simulated Universe Hypothesis
It is intriguing and unnerving to consider the possibility that our universe is not “real” but rather a very complex simulation. Philosophers, scientists, and technologists have all been captivated by this theory, which has sparked discussions about the nature of reality and the role of humans in it.
Could our lives, our planet, and even the stars themselves be part of an elaborate computational construct? Here we will dive deeply into the simulation hypothesis, examine its connection to the holographic principle, and explore clues from black holes and modern physics.
What Is the Simulation Hypothesis?
The simulation hypothesis posits that reality as we perceive it might be an advanced simulation created by a highly sophisticated civilization. Philosopher Nick Bostrom brought this idea to mainstream attention in his 2003 paper, Are You Living in a Computer Simulation? Bostrom outlines a trilemma:
- Civilizations never reach the level of technological advancement needed to create simulations.
- Advanced civilizations choose not to run simulations.
- If simulations are possible and chosen, we are almost certainly living in one.
The last point hinges on probability. If one civilization could run countless simulations of universes, the simulated worlds would vastly outnumber the real one. By this logic, itโs statistically more likely we are living in a simulated universe than a real one.
Black Holes: Cosmic Clues to a Simulated Universe

The Firewall Paradox and Fuzzball Theory
Black holes, long considered cosmic destroyers, have undergone a theoretical makeover in recent years. Traditionally, scientists thought of black holes as dense regions where gravity is so strong that not even light can escape. However, this view clashed with the laws of quantum mechanics, which assert that information cannot be destroyed.
This conflict gave rise to the firewall paradoxโa theory suggesting that anything crossing a black holeโs event horizon would meet a fiery end.
Enter the fuzzball theory, proposed by physicist Samir Mathur. This groundbreaking idea suggests that black holes are not voids but rather tangled masses of cosmic strings. Instead of destroying matter, black holes store it in a “fuzzball,” preserving its information. This resolves the information paradox and paints black holes as less destructive than previously thought.
Holograms on the Event Horizon
Mathurโs fuzzball theory ties closely to the holographic principle, which argues that all the information contained in a 3D space can be encoded on its 2D surface. Black holes exemplify this principle: when matter enters a black hole, its information is projected onto the event horizon, forming an imperfect hologram.
This imperfection is critical. Physicist Stephen Hawking emphasized the universeโs inherent flaws, noting that black holes, like the cosmos itself, are not perfect but unique. These imperfections might explain how information is preserved, even in the extreme environment of a black hole.
The Holographic Principle: A Radical Shift in Understanding
The holographic principle challenges the way we perceive reality. Initially rooted in black hole physics, the principle suggests that our three-dimensional universe could be a projection of information encoded on a distant two-dimensional surface. Think of a hologram on your credit card: a 2D image creates the illusion of depth and three-dimensionality.
This concept gained traction in the 1990s, thanks to physicists Gerard ‘t Hooft and Leonard Susskind. They proposed that the holographic principle applies not just to black holes but to the entire universe. If true, it would mean that our 3D reality is merely an illusionโa shadow cast by information stored elsewhere.
Could Dark Energy Be the Key?
Dark energy, the mysterious force driving the accelerated expansion of the universe, might offer clues about the holographic nature of reality. Some physicists believe that dark energy arises from the holographic properties of the cosmos. In this model, the universeโs expansion could eventually lead to its “end”โeither through a big rip, where galaxies, stars, and atoms are torn apart or a heat deathย where everything freezes into stillness.
This connection between dark energy and holography adds another layer to the simulation hypothesis. If the universeโs expansion is governed by information encoded on a 2D boundary, it could imply that the cosmos itself is a computational construct.
Advanced Civilizations: The Architects of Simulated Universes?
For the simulation hypothesis to hold, there must be civilizations capable of creating such simulations. These beings would need computational power far beyond anything humanity has achieved. But why would they create simulations in the first place? Several motivations have been proposed:
- Scientific Curiosity: Simulating universes could help advanced civilizations study the origins of their own existence or test theories about physics and evolution.
- Entertainment: Just as humans create video games, advanced beings might build simulations for leisure or artistic expression.
- Ethical Considerations: Some argue that creating simulations to house conscious beings could raise moral dilemmas, potentially deterring advanced civilizations from doing so.
Critiques and Challenges to the Simulation Hypothesis
Despite its allure, the simulation hypothesis faces significant challenges. One major critique is the sheer complexity of simulating an entire universe. Such a task would require not only immense computational power but also a complete understanding of the laws of physics.
Additionally, skeptics question whether simulated beings could truly experience consciousness. Could a computer program replicate emotions, creativity, and free will? This debate touches on deep philosophical questions about the nature of mind and self-awareness.
Another point of contention is the “problem of counting simulated brains.” Bostromโs argument assumes that simulations would outnumber real worlds. However, we lack concrete evidence about the prevalence of advanced civilizations, let alone their interest in creating simulated universes.
The Connection Between Physics and Philosophy
The simulation hypothesis is as much a philosophical thought experiment as it is a scientific idea. It echoes centuries-old questions about reality, perception, and existence. Ancient philosophers like Plato wrestled with similar ideas, as seen in his Allegory of the Cave, where shadows on a wall represent a distorted version of reality.
Modern physics adds a new dimension to these discussions. Concepts like the holographic principle, quantum mechanics, and dark energy suggest that the universe is far stranger than we ever imagined. Whether or not we live in a simulation, these ideas challenge us to expand our understanding of what is possible.
Mathematical Evidence for Simulation: Exploring the Universe as a Code
One of the most intriguing aspects of the simulation hypothesis is the possibility that the universe itself operates on mathematical structures resembling computer code. This idea suggests that our reality might be underpinned by binary systems, algorithms, or other computational frameworks. From the patterns in physics to the nature of reality at the smallest scales, mathematics could be the “language” of the universeโor evidence that it was coded by advanced beings.
The Universe as a Mathematical Construct
Mathematics has long been described as the “universal language.” It underpins the laws of nature, from the motion of planets to the behavior of particles. Many scientists and philosophers, including Galileo and Einstein, have marveled at how abstract mathematical principles describe the real world with astonishing accuracy. Could this uncanny alignment suggest that the universe is designedโor programmed?
Physicist Max Tegmark argues that the universe is not just described by mathematics but is mathematics. His Mathematical Universe Hypothesis posits that everything in existence corresponds to a mathematical structure. In this view, physical reality is merely a manifestation of mathematical relationships, similar to how a computer simulation runs on binary code.
Binary Systems: The Universe as a Digital Construct
One of the most compelling arguments for a simulated universe lies in the idea of binary systems. Computers operate on a binary code of 0s and 1s, using these simple units to create complex programs, images, and virtual realities. Similarly, at the quantum level, reality appears to exhibit binary-like behavior.
For instance:
- Quantum Bits (Qubits): In quantum computing, information is encoded in qubits, which can exist in states of 0, 1, or both simultaneously (superposition). This mirrors the dual nature of particles in quantum mechanics, which can behave as both particles and waves.
- Discrete Nature of Reality: Many physicists believe that space, time, and energy are quantized, meaning they come in discrete units rather than being continuous. This is akin to pixels in a digital image, where reality is divided into the smallest possible “chunks.”
These parallels raise the question: could the universe itself be running on a binary framework, much like a computer?
Algorithms and the Fabric of Reality
Another key aspect of the simulation hypothesis is the role of algorithmsโstep-by-step computational instructions. In the natural world, algorithms appear in surprising places:
- Genetic Code: DNA operates like a biological algorithm, using sequences of nucleotides to encode instructions for building life.
- Fractals: Complex patterns found in nature, like snowflakes or coastlines, can be described by simple mathematical rules repeated at different scales. These resemble iterative processes in programming.
- Fibonacci Sequence: This mathematical sequence, where each number is the sum of the two preceding numbers, appears in phenomena ranging from the arrangement of leaves to the spirals of galaxies.
These patterns suggest that algorithms may govern the universeโs structure and behavior. If so, they could be evidence of an underlying computational design.
Digital Physics: The Universe as a Simulation
The field of digital physics takes these ideas a step further, proposing that the universe operates like a giant computer. Physicist Konrad Zuse was one of the first to suggest this concept, arguing that the cosmos might function on a cellular automatonโa grid of cells that evolve based on specific rules. Cellular automata have been used to model complex systems, from ecosystems to galaxies, reinforcing the idea that reality could be algorithmic.
In 2023, physicist Melvin Vopson proposed the Second Law of Infodynamics, which states that information may be a fundamental building block of the universe. He speculated that information entropy, akin to data processing, decreases over time, supporting the notion of a computational universe. This aligns with the simulation hypothesis, as it suggests the universe’s behavior could be optimized like a computer program.
The “Code” Found in Physical Constants
Perhaps the most tantalizing clue comes from the apparent fine-tuning of physical constants. For example:
- The speed of light, the gravitational constant, and the strength of the electromagnetic force all have precise values that allow life to exist.
- If these constants were even slightly different, stars might not form, atoms could collapse, or life might never emerge.
The improbability of such perfect calibration has led some scientists to wonder if these constants were “programmed” into the universe, much like a software developer fine-tunes a simulation.
Additionally, theoretical physicist James Gates discovered what he called “error-correcting codes” embedded in the equations governing supersymmetryโa potential framework for unifying all forces in physics. These codes resemble those used in computer programming to detect and fix errors, adding weight to the idea that reality might be computational.

Criticisms and Counterarguments
While the mathematical elegance of the universe is compelling, critics caution against jumping to conclusions. They argue that:
- The universeโs mathematical nature could be coincidental or a reflection of human cognition, which relies on mathematics to make sense of the world.
- Concepts like fine-tuning might arise from the anthropic principle, which states that we observe the universe as it is because we exist within it. If the constants were different, we wouldnโt be here to notice them.
- Digital physics remains speculative, lacking experimental evidence to prove the universe operates on computational principles.
Implications of a Mathematical Universe
If the universe is indeed a computational construct, it raises profound questions:
- Who or what created it?: Is the universe the product of an advanced civilization, a natural phenomenon, or even a cosmic accident?
- Can we “hack” the system?: If reality operates on algorithms, could we one day manipulate them to reshape the universe?
- What is consciousness?: If we are part of a simulation, is our awareness genuine, or is it an emergent property of complex programming?
These questions stretch the boundaries of science and philosophy, inviting us to rethink what it means to exist.
What Does It All Mean?
If the simulation hypothesis is true, it fundamentally changes our perspective on life and the universe. However, it doesnโt diminish the significance of our experiences. Whether real or simulated, our joys, struggles, and achievements remain meaningful. As physicist Paul Sutter notes, “You live your life the same way, whether it’s real or not.”
Ultimately, the simulation hypothesis invites us to think more deeply about the nature of reality. Are we the architects of our own destiny, or are we players in a cosmic game? Either way, the pursuit of knowledge continues to inspire humanity to reach for the starsโreal or simulated.
The idea that the universe operates on code-like structures blurs the line between physics and metaphysics. From quantum bits and fractals to fine-tuned constants and error-correcting codes, evidence mounts that reality might be more computational than we ever imagined. While we may never know for sure if we live in a simulation, exploring the mathematical underpinnings of the cosmos deepens our appreciation for the mysteries of existenceโand our quest to unravel them.
Dark Energy Cast of Characters
Albert Einstein (1879 – 1955): A theoretical physicist who assumaly revolutionized our understanding of space, time, and gravity. He developed the theories of special and general relativity, introducing the concept of spacetime and proposing that gravity is the curvature of spacetime. He also introduced the cosmological constant, initially to explain a static universe but later rejected it as a blunder. However, with the discovery of dark energy, his concept has been revisited.
Isaac Newton (1643 – 1727): A physicist and mathematician who laid the foundations of classical mechanics and described gravity with remarkable accuracy. He viewed space as a static and absolute framework, a stage on which the events of the universe unfold.
Hendrik Casimir (1909 – 2000): A Dutch physicist known for predicting the Casimir effect, which demonstrates that “empty space” at the quantum level is not truly empty but filled with particle fluctuations that can exert a measurable force.
Samir Mathur: A professor of physics at Ohio State University known for his “fuzzball theory” of black holes. This theory, rooted in string theory, proposes that black holes are not empty voids but rather dense, tangled balls of cosmic strings that act as “hologram generators” when matter interacts with them.
Leonard Susskind (born 1940): A theoretical physicist at Stanford University, considered one of the fathers of string theory. He is known for his work on black hole information paradox, suggesting that information is not lost when it enters a black hole but is encoded on the event horizon, and also for proposing the holographic principle.
Stephen Hawking (1942 – 2018): A renowned physicist and cosmologist known for his work on black holes, the Big Bang, and the nature of space and time. He initially argued that information is lost in black holes but later conceded that it might be preserved, contributing to the resolution of the “information paradox.” He also famously stated that the universe was imperfect from its initial moments.
Leonard Schiff (1915 – 1971): An American physicist who, along with William Fairbank and Robert Cannon, conceived of Gravity Probe B, a groundbreaking experiment to test Einstein’s prediction that Earth’s rotation drags spacetime.
William Fairbank (1917-1989): An American physicist who played a key role in the development of Gravity Probe B. He was particularly focused on developing the incredibly smooth gyroscopes needed for the experiment.
Robert Cannon (1931 – 2000): An American physicist and engineer who was a key member of the Gravity Probe B team, contributing to the design and implementation of the experiment.
Peter Higgs (born 1929): A British physicist known for his theoretical prediction of the Higgs boson and the Higgs field, which explains how particles acquire mass. The discovery of the Higgs boson at CERN in 2012 confirmed his theory and significantly advanced our understanding of the nature of space.
Nick Bostrom (born 1973): A philosopher at Oxford University known for his work on existential risks, transhumanism, and the simulation hypothesis. He argues that if humans can create highly realistic universe simulations, it’s statistically probable that we are already living in one.
Paul M. Sutter: An astrophysicist and science communicator known for his YouTube channel and podcast “Ask a Spaceman!” He has discussed the simulation hypothesis in detail, outlining both the arguments in favor of it and the challenges it faces, including the computational limitations of simulating an entire universe and the question of whether simulated consciousness is truly equivalent to organic consciousness.
Melvin Vopson: A physicist at the University of Portsmouth who proposed the second law of infodynamics, suggesting information is a fundamental building block of the universe and that information entropy decreases over time. He believes this law could provide evidence for the simulation hypothesis, as the universe’s tendency towards order might reflect the optimization processes of a computer program.
Serban Lepadatu: A mathematician at the Jeremiah Horrocks Institute for Mathematics, Physics and Astronomy in the UK who collaborated with Melvin Vopson on the development of the second law of infodynamics. Their work explores the implications of this law for various scientific disciplines, including genetics, cosmology, atomic physics, and the simulation hypothesis.
Event Timline
This timeline combines information from all sources to map a progression of understanding related to the nature of space:
1687:
- Isaac Newton publishes “Principia Mathematica,” describing space as a passive, absolute, eternal, and unchanging stage for the universe.
Late 1800s:
- Albert Einstein is captivated by the nature of light, particularly its speed.
Early 1900s:
- Einstein develops the theory of special relativity, proposing that space and time are flexible and interconnected, forming a single entity called spacetime.
- Einstein publishes his theory of general relativity, proposing that gravity is not a force but the curvature of spacetime caused by massive objects.
1948:
- Hendrik Casimir predicts the Casimir effect, demonstrating that “empty space” on atomic scales is not empty but teeming with particle activity.
1959:
- Leonard Schiff, William Fairbank, and Bob Cannon conceive of Gravity Probe B, an experiment to test Einstein’s prediction that Earth’s rotation twists spacetime.
1962:
- The Gravity Probe B team applies for a NASA grant.
1964:
- Peter Higgs proposes the Higgs field, suggesting that particles gain mass through their interaction with this field that permeates space.
Sources for Further Reading
- Nick Bostromโs Original Paper: Simulation Argument
- ScienceDaily: Black Holes as Holograms
- IFLScience: Holographic Dark Energy
Sources for Mathematical Evidence for Simulation
Here are some notable sources discussing mathematical evidence and theories related to the simulation hypothesis:
- Nick Bostromโs Paper: Are You Living in a Computer Simulation?
Bostrom outlines the statistical basis for the simulation hypothesis.
Link - Max Tegmarkโs Work: Our Mathematical Universe
This book explores the idea that mathematical structures are not just tools to describe reality but the very fabric of existence.
Link - James Gatesโ Discovery of Error-Correcting Codes
Physicist James Gates found binary error-correcting codes in supersymmetry equations, which resemble those used in computer programming.
Popular Science Article - Melvin Vopsonโs Second Law of Infodynamics
Vopson proposed that information is a fundamental building block of the universe, and his work supports the idea of a computational reality.
Research Summary - Konrad Zuseโs Cellular Automaton Theory
Zuse proposed that the universe might operate on a computational framework akin to a cellular automaton.
Reference Overview